Astronomy and Astrophysics
Haystack's astronomy and astrophysics research program is diverse; the Observatory's staff members explore the origin of the first stars in the universe, black holes in nearby and distant galaxies, the birth and death of stars, and several other areas of cosmic physics. All of this research is driven by the Observatory's expertise in carrying out cutting-edge observations at radio wavelengths.
On these pages we describe general research topics in astronomy and astrophysics that are currently being explored by Haystack scientists. For information on specific research projects being conducted by the Astronomy group, see the Astronomy projects page.
Black Hole Physics
Black holes pack an incredibly large mass into an unimaginably small volume. Supermassive black holes, found in galactic centers, can have a mass of more than ten billion times that of the Sun. The singularity is not directly observable, being shrouded by an event horizon from which even light cannot escape. Nevertheless, the region around the event horizon hosts the most extreme gravitational fields to be found in the universe. Black holes thus serve as an ideal laboratory for testing general relativity in the strong-field regime.
Supermassive black holes are among the most powerful engines in the universe, with effects felt far beyond the region in which they are gravitationally dominant. Supermassive black holes in distant galaxies can launch powerful relativistic jets that extend beyond the host galaxy itself. Feedback from these jets is dynamically important to both star formation and galaxy cluster evolution over cosmic time.
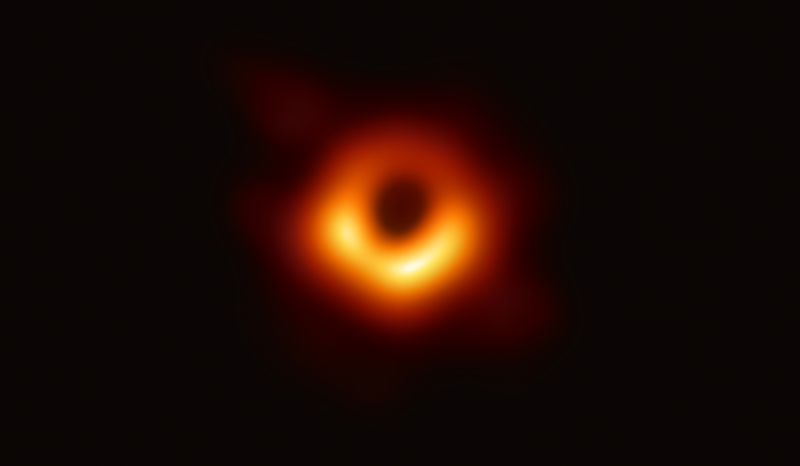
Related Projects
Researchers at Haystack study two particular aspects of black hole physics:
- The Event Horizon Telescope project connects telescopes around the globe during short campaigns of a few days per year to resolve the event horizons of relatively nearby supermassive black holes. The goal of this work is to probe the fundamental physics and astrophysics of black holes, including the processes of accretion and the launching of outflows.
- The ALMA Phasing Project (APP) is an international project led by Haystack Observatory to turn the ALMA Observatory in northern Chile into the world’s most sensitive millimeter-VLBI station.
- Investigations with other telescope arrays allow investigation of the overall demographics of black holes.
Researchers
Star Formation
Stars continue to form in the Milky Way and other galaxies. A prominent example for a relatively nearby star-forming region (i.e, about 1,500 light-years away) is the Orion Nebula, which can be seen with the naked eye.
Stars, and the planets orbiting them, form at the center of collapsing clouds of cosmic gas. This gas contains numerous molecules, and these molecules emit radiation that can be detected at radio wavelengths. It is therefore possible to explore star-forming gas very efficiently with radio telescopes. Many aspects of star formation can, in fact, be studied only at radio wavelengths.
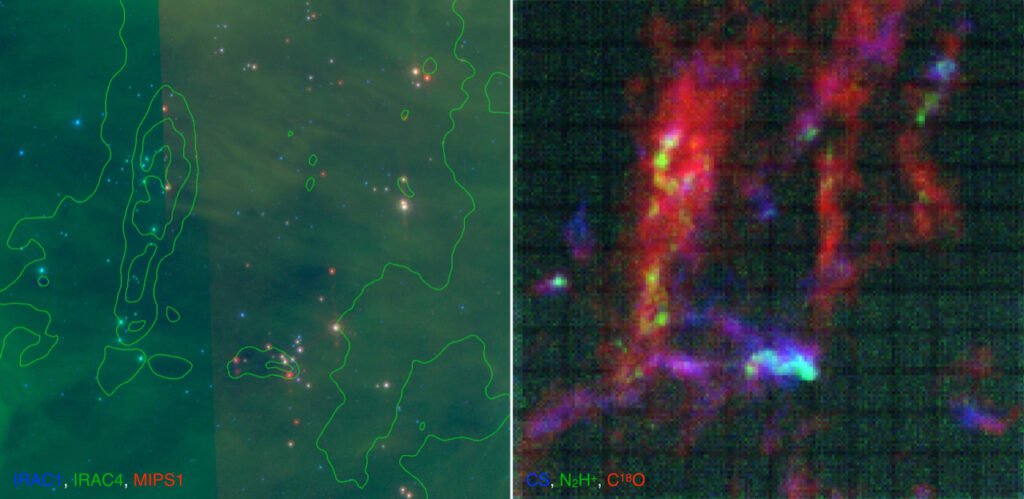
Haystack’s research into star formation focuses on using radio telescopes to determine the physical parameters of star-forming molecular clouds, such as their mass, their density, and the characteristics of internal gas motions (e.g., turbulence and collapse motions). Observations of molecular abundances also allow to assess the “age” of molecular gas, as the astrochemical composition of cosmic gas changes over time. These measurements constrain theoretical models of star formation that are used to explain, for example, the rate at which stars form in the Milky Way and other galaxies.
Related Projects
The LEGO survey of molecular clouds in the Milky Way images 25 molecular clouds in various emission lines near 100 GHz frequency to generate a representative atlas of molecule distributions in star-forming regions. This atlas is used to test and develop tools that allow to gauge the gas conditions in star formation regions in other galaxies.
Researchers
Radio Stars
Detectable radio emission arises from stars spanning virtually every type and evolutionary stage. Stellar radio emission may originate from a wide variety of physical processes, including free-free emission from ionized winds and coronae, gyrosynchrotron radiation, atomic and molecular emission lines, radio recombination lines, and electron cyclotron masers.
The observation of stars in the radio (i.e., at wavelengths ranging from meter to submillimeter) provides wide-ranging insights into the lives of stars. Radio observations can be used to measure fundamental stellar parameters (e.g., size, mass, temperature, distance, magnetic field) and they provide unique information on how stars are born, evolve, interact with their environments, and eventually die.
Haystack scientists are involved in several ongoing programs that utilize radio wavelength observations to address key questions in stellar astrophysics. Some examples are described below. Haystack Observatory has also hosted two international workshops on “Radio Stars” (in 2012 and 2017) and has developed a “Radio Stars” podcast series to share results with the general public.
Related Projects
Stellar Imaging: using radio interferometers to image the dynamic surfaces of asymptotic giant branch (AGB) stars and red supergiants.
Mass Loss from Red Giants: surveys of AGB stars and red supergiants in the HI 21-cm line to probe stellar mass-loss histories and how dying stars interact with their interstellar environments.
During the late stages of their evolution, most stars undergo periods of intensive mass loss, shedding up to 80% of their initial mass through intense stellar winds. The result is the formation of enormous circumstellar envelopes that can span up to several light years across and serve as a fossil record of the mass loss history of the star. The debris shed by dying stars also enriches the interstellar medium with dust, heavy elements, and molecules, and ultimately seeds the formation of the next generation of stars.
In many of the evolved stars observed in the HI 21-cm line to date, the properties of the hydrogen envelopes are found to be strongly influenced by the interaction between the and the interstellar medium (ISM). Stars within our Galaxy are not stationary, but rather are moving through the ISM with typical velocities of ~10-100 km/s. This results in distortion of the circumstellar shells, and in some cases leads to the formation of spectacular comet-like tails (see examples below).
The rich chemistry and cool temperatures of circumstellar envelopes give rise to a multitude of molecular emission lines that can be observed at radio wavelengths. However, Haystack researchers have also been using an alternative approach to study circumstellar environments: observations of the 21-cm line emitted by the neutral hydrogen atom (HI). Unlike most molecules, HI atoms in the outskirts of the circumstellar envelopes are not dissociated by the interstellar radiation field. Observations of circumstellar HI are capable of tracing the distribution and kinematics of ejecta to much larger distances from the star, thereby tracing a much larger fraction of the star’s mass-loss history—up to a million years or more.
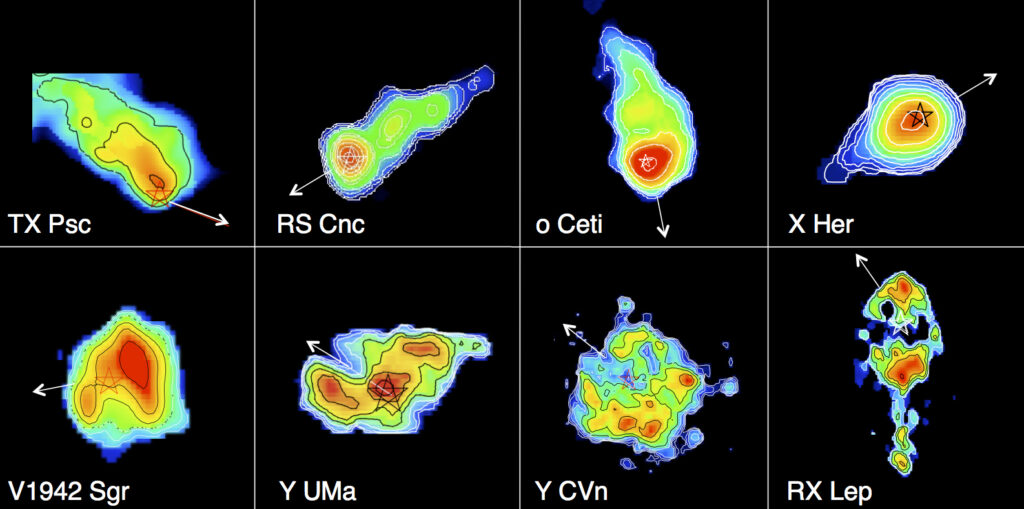
Stellar Masers: studies of SiO, H2O and OH masers in the atmospheres of evolved stars and massive young stellar objects to probe their dynamic atmospheres.
The Secrets of Cepheids: Using radio continuum and HI 21-cm line observations to search for evidence of mass loss from Cepheid variable stars.
Cepheid variables are a type of star that undergoes regular radial pulsations, typically with periods of order of several days. Cepheids are of fundamental importance to astronomers, because there exists a tight correlation (known as the “P-L relation” or “Leavitt Law”) between the luminosity of these stars and their pulsation period. This relation allows astronomers to straightforwardly determine the distances to Cepheids, even those that reside in other galaxies. Cepheids thus serve as “standard candles” that allow astronomers to determine the distances to galaxies throughout the local universe.
However, despite their importance to astronomy, Cepheids still pose many unsolved puzzles. One of the most vexing is the so-called “Cepheid mass discrepancy”—a persistent 10–15% disagreement between the masses of Cepheids inferred from stellar evolution models compared with those derived from the Leavitt Law.
A long-proposed solution to the Cepheid mass discrepancy is that Cepheids are undergoing high rates of mass loss. But despite decades of searches, direct observational evidence of Cepheid mass loss has remained elusive—until recently. This has begun to change, thanks to several new types of observations, including some carried out by Haystack researchers using radio telescopes.
One approach pioneered by Haystack researchers involves searching for circumstellar ejecta surrounding Cepheids using observations of the 21-cm line of neutral atomic hydrogen (HI). Studies of Cepheids in the HI line using the Karl G. Jansky Very Large Array in New Mexico has led to the detection of an enormous hydrogen nebula surrounding the Cepheid archetype δ Cephei , providing compelling evidence of significant mass loss. The more massive Cepheid T Mon also appears to have an associated HI envelope that likely originated from mass loss.
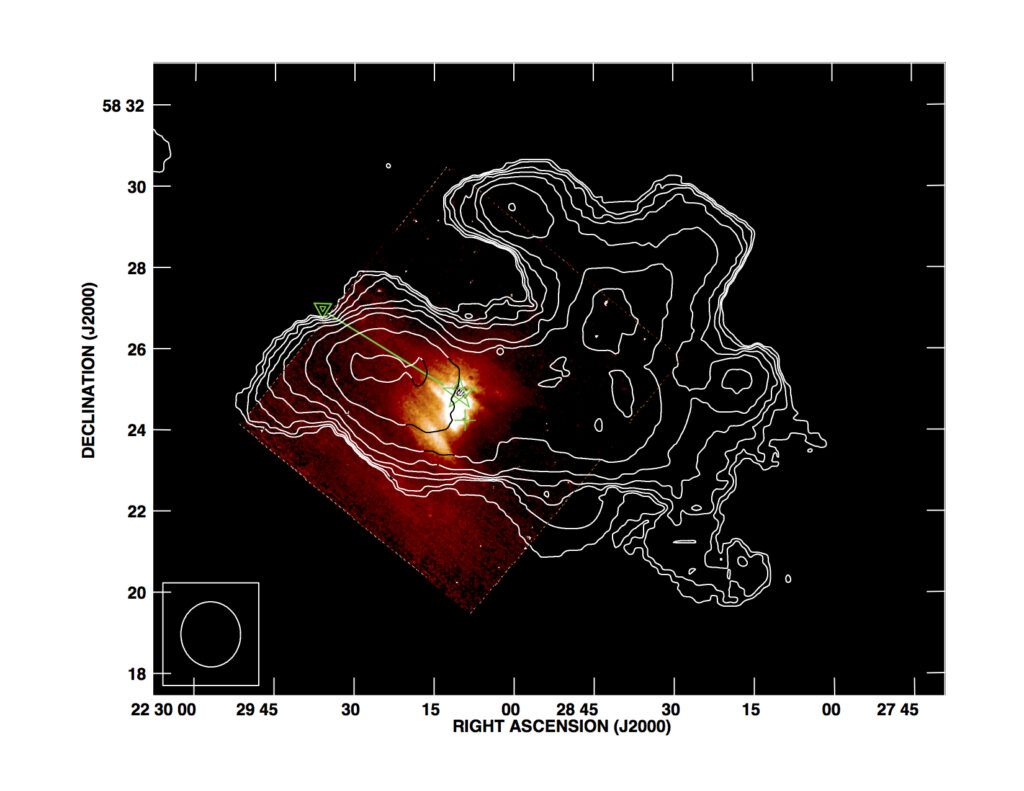
A second approach to searching for evidence of Cepheid mass loss at radio wavelengths involves deep observations of Cepheids in the radio continuum at ν~15 GHz. If Cepheids are losing mass through stellar winds that become partially ionized as a result of shocks, the winds will then emit radio free-free emission. Haystack researchers have recently obtained the first ever detection of radio continuum emission from a Cepheid.
Solar Imaging: imaging the Sun and solar corona at low radio frequencies.
The nearest radio star is our own Sun. At low radio frequencies, the solar corona glows brightly due to its high temperature, and dynamic magnetic structures give rise to intense emissions of several different types. Using the Murchison Widefield Array (MWA) in Western Australia, that Haystack Observatory helped to design and build, solar images are obtained with unprecedented dynamic range revealing hitherto unsuspected phenomena.
Researchers
- Lynn Matthews
- Kazunori Akiyama
- Leonid Benkevitch
- Colin J. Lonsdale
Epoch of Reionization
The Epoch of Reionization (EOR) is the period in the history of the universe during which the predominantly neutral intergalactic medium was ionized by the emergence of the first luminous sources. These sources may have been stars, galaxies, quasars, or some combination of the above. By studying reionization, we can learn a great deal about the process of structure formation in the universe, and find the evolutionary links between the remarkably smooth matter distribution at early times revealed by studies of the cosmic microwave background (CMB), and the highly structured universe of galaxies and clusters of galaxies at redshifts of 6 and below.
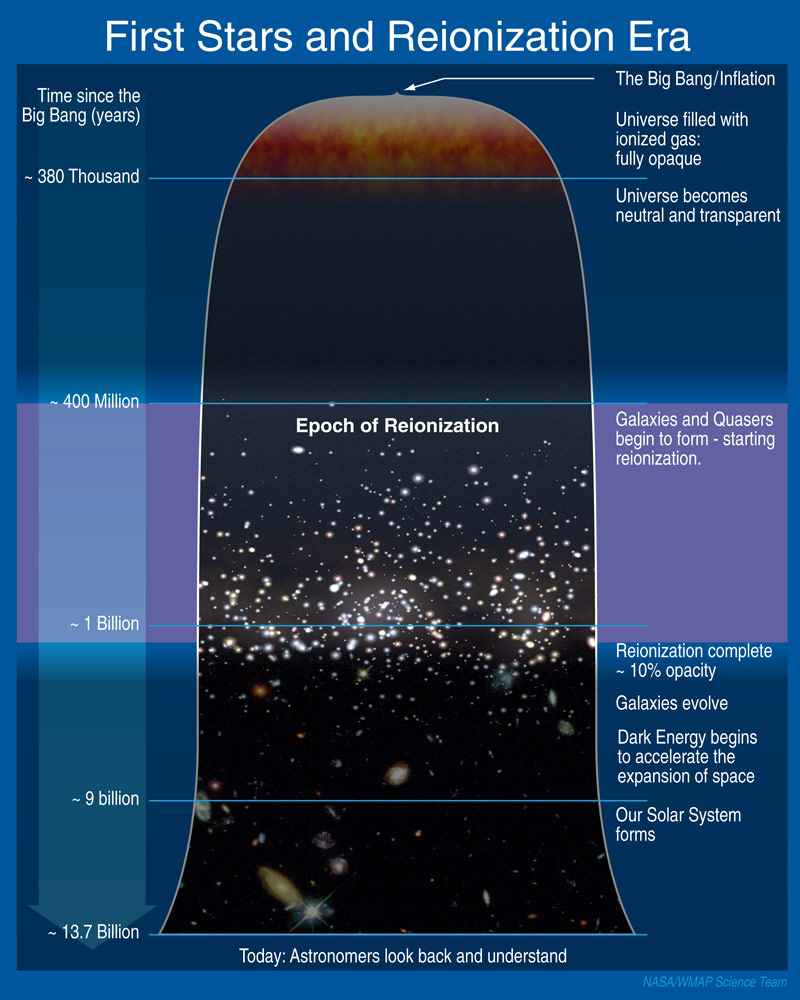
This diagram provides a good graphical representation of the history of the universe, and where the epoch of reionization sits in the overall picture. After the Big Bang, the universe was a hot but quickly cooling soup of fundamental particles. After a few hundred thousand years, things cooled enough so that protons and electrons could combine to form neutral hydrogen. This was a rather sudden event, and allowed the thermal glow of the fireball plasma, as it existed immediately before the hydrogen formation event, to radiate throughout the universe unimpeded by constant interactions with the charged particles of the now-absent plasma. This glow, redshifted by a factor of 1100 or so, is what we now observe as the cosmic microwave background (CMB) in all directions. The CMB carries a frozen imprint of the density fluctuations in the early universe, the study of which, by the observational cosmology community, is intense and sustained.
After the universe became neutral, it became unobservable across much of the electromagnetic spectrum. Any short wavelength radiation that might have been emitted was quickly absorbed by the atomic gas, and a long interval known as the Dark Ages began. Slowly, gravitational collapse of over-dense regions, the same regions we can see in the CMB imprint from earlier times, led to the formation of more and more pronounced structure in the neutral medium, and eventually the first stars, galaxies and quasars started to form. The exact mechanism and nature of this formation, poorly constrained by observation, is a topic of much research and great importance. We know what the universe looked like at the time of the CMB, and we know what it looks like now, but how did it get from one to the other?
As the collapse of structures proceeded, temperature variations developed. Gradually, energetic radiation emitted by the first sources caused local heating, and then ionization of the hydrogen in the universe. It will have started with “bubbles” of ionized plasma surrounding the most energetic sources. As the bubbles grew and became more numerous, they started to overlap, and more and more of the neutral medium became exposed to the harsh ionizing radiation, which travels unimpeded through ionized regions. The final phase of reionization of the universe may have occurred swiftly. As soon as the bulk of the universe was reionized, light at many wavelengths could escape from the early galaxies and quasars, revealing the distant universe that we see today with optical and infrared telescopes.
Reionization was complete about 1 billion years after the Big Bang, corresponding to a redshift of about 6.5. Before that time, observations rapidly become more difficult. By and large, one must hope to find isolated, very luminous objects whose radiation in one form or another manages to reach us through the increasingly neutral medium. Perhaps the best hope for a more general and comprehensive probe of these early epochs is the 21cm hyperfine transition line of neutral hydrogen, redshifted to frequencies below 200 MHz. Sensitive observations of emission and absorption in this line can probe deeply into the reheating and reionization epochs, and give us a detailed view of the density, temperature, and velocity field of the material. We would get a view, not just of isolated luminous objects and the material which happens to lie in front of them, but of large volumes of the universe at the target redshifts. Such a view would yield a treasure trove of information from which to deduce the early history of structure formation, and the origin of the stars, galaxies, clusters, and quasars that we see today.
Related Projects
The EDGES project was specifically designed to detect EoR signatures. The detector systems fielded by this project deliver data of unprecedented quality at frequencies between about 50 and 200 MHz.
Researchers
Very Long Baseline Interferometry (VLBI)
Telescopes must be large in order to resolve fine detail in celestial objects. The sizes of telescopes are, however, in practice limited due to constraints in engineering and budgets. Very long baseline interferometry (VLBI) allows to partially overcome these constraints by forming “virtual” telescopes that can have a size larger than the diameter of Earth.
To do this, VLBI connects arrays of several telescopes of limited size. The resulting synthesized telescope achieves an angular resolution driven by the largest separation between the telescopes within the array. Provided the array is Earth–bound, separations equal to Earth’s diameter can be achieved. Some VLBI experiments also include telescopes in space, thus allowing to achieve telescope separations larger than Earth’s diameter.
The connections within a VLBI network are formed “offline,” that is, by processing recorded telescope data well after the end of observations. To do this, the stations within a VLBI array write the telescope data onto very fast hard disks, with incredibly precise timestamps provided by maser clocks. These disks are then physically shipped to a research institute hosting a “correlator,” which is a fast computer that can jointly process the data from several VLBI stations in order to synthesize a virtual telescope. The correlator at Haystack Observatory, for example, is used to process data from the Event Horizon Telescope project and the VLBI Global Observing System (VGOS).
VLBI is a well-established technology with a long history. It was developed in the 1960s, with Haystack Observatory playing a central role in creating this technique. VLBI technology continues to evolve. Current engineering projects focus, for example, on recording VLBI telescope data at ever-increasing rates, and on making VLBI techniques work at frequencies well above 100 GHz. Haystack continues to play a world-leading role in these activities.
Common terms
baseline
A baseline is the distance between two VLBI antennas. The pair of antennas are often very far apart—in some cases, thousands of kilometers. Longer baselines increase the sensitivity of the measurements.
interferometry
Interferometry is a technique that uses pairs of radio antennas. The radio antennas, or telescopes, observe the same distant source at the exact same time. Their signals are superimposed (correlated) in a mathematical process that makes use of interference. In astronomy, interferometry is used to image distant objects. In geodesy, interferometry is used to measure the earth.
correlator
At correlator, the signals from many radio telescopes around the globe are combined, or correlated, using interferometry. The result of using signals from a pair of telescopes gives more information than the signal from either single telescope.
radio waves
Radio waves are a type of electromagnetic (EM) radiation. Everything in the universe emits EM radiation, including celestial objects. Measuring the radio signal or radio waves from objects in space allows us to obtain an enormous amount of information about these objects.
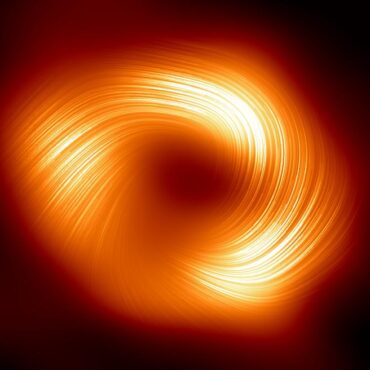
Black Hole Explorer (BHEX)
A new mission to measure a black hole's photon ring, capturing light orbiting a black hole.
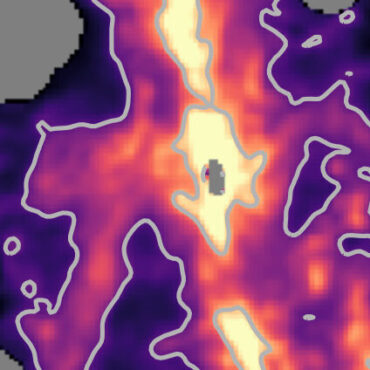
LEGO
LEGO studies star-forming sites in the Milky Way in order to better understand similar regions in nearby galaxies. It uses the most comprehensive wide-field atlas of molecules in space to do so.
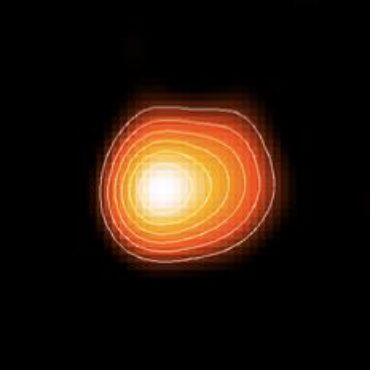
Radio Stars
All stars emit radio waves. The study of stellar radio emission provides unique insights into the lives of stars that cannot be obtained by observations at other wavelengths.