Geospace & Atmospheric Science
Selected topics from the group's wide-ranging investigations of geospace morphology and dynamics in near-Earth space are available on these science tabs. Areas of particular emphasis are on the charged upper atmosphere from ~90 km altitude out to tens of earth radii (ionosphere and plasmasphere) and the neutral upper atmosphere where atoms and molecules gradually separate by mass as a function of altitude (thermosphere). Also crucially important is tight electrodynamic coupling, connecting the charged electrons and ions forming the ionosphere to the near-Earth regions of space controlled by the strong background magnetic field (magnetosphere). This tightly woven, interlocked system has both long-term trends (climate) and short-term variations (space weather) on scales from tiny to vast, in both space and time.
More on these areas of research in the science tabs below.
Whole-Atmosphere Coupling
Haystack researchers study the coupling of atmospheric layers across a wide scale, from troposphere and stratosphere to mesosphere and thermosphere/ionosphere. We often use large-scale dynamics events like sudden stratospheric warming to understand how different layers of our atmosphere are connected with each other.
A sudden stratospheric warming (SSW) is one of the most radical changes of weather observed on our planet, and produces a number of intriguing atmospheric features. Within several days, North Pole temperatures can increase by more than 50 K (90ºF) in an SSW event. Several years ago, Haystack researchers have discovered that contrary to previous ideas, the effects of sudden stratospheric warmings do not merely stay in the stratosphere. Instead, large and dramatic disturbances are also observed far above the stratosphere. These changes can affect atmospheric chemistry, temperatures, winds, neutral (nonionized particle) and electron densities, and electric fields, and they extend from the surface to the thermosphere and across both hemispheres. These changes span regions that scientists had not previously considered to be connected.
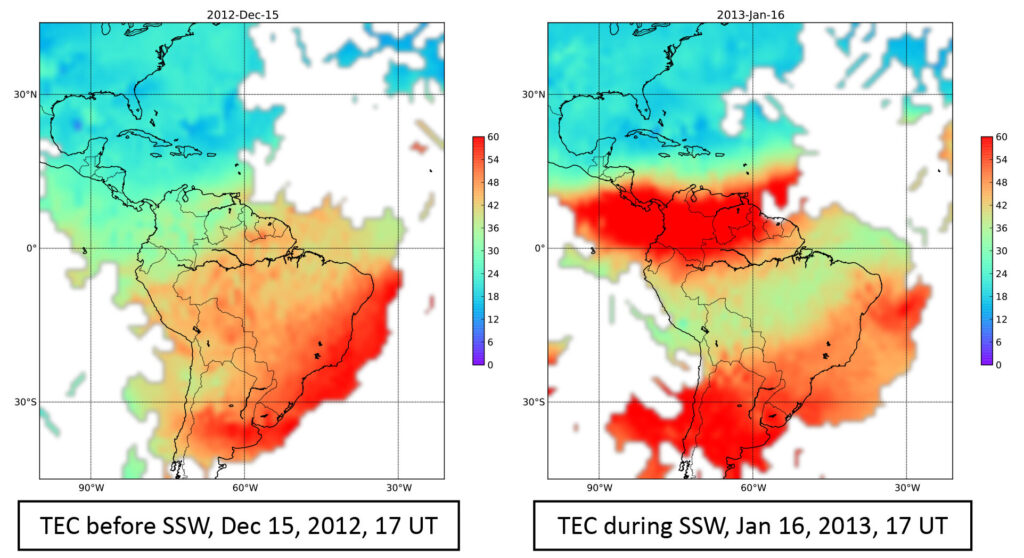
Understanding these coupling mechanisms has practical importance: SSWs open the door for improved tropospheric and space weather forecasting capabilities. The implications extend not only to weather forecasting here on the surface but also to greater understanding of chemical processes in the atmosphere, the sources of adverse effects on satellite navigation systems (e.g., GPS) and telecommunications, and possibly even the study of atmospheres on other planets.
Geospace Storm and Disturbance Studies
Geospace studies at Haystack include an active focus on how dynamic solar activity impacts near-Earth space, with a particular concentration on physical processes of magnetosphere-ionosphere-thermosphere coupling that occur in response to these enormous energy inputs. We use a system science approach to examine dynamics of these regions in the mid-latitude and sub-auroral regions. Haystack observations that are particularly well suited for these studies include the NSF-sponsored incoherent scatter radar measurements at Millstone Hill, and GNSS global TEC data.
Studies in these areas are particularly influenced by the significant effects of magnetosphere-ionosphere coupling. The planet’s strong background terrestrial magnetic field provides an important long-range force that combines with several influences: strong binding of charges to this field; dynamo effects as neutrals move across the magnetic field creating electric fields; and ion-neutral collisions that couple ion motions to neutrals and vice-versa at selected altitudes. These factors mean that electric and magnetic fields far out into near-Earth space can have profound consequences for the neutral and ionized atmosphere much closer to the ground, and can radically alter the configuration of these regions in both space and time.
We work with research community members, including modelers, to make new discoveries and to enhance fundamental understanding of solar-terrestrial physics. Such research is an important part of community efforts to provide space weather prediction capability, and is a crucial task as humans become an increasingly spacefaring species.
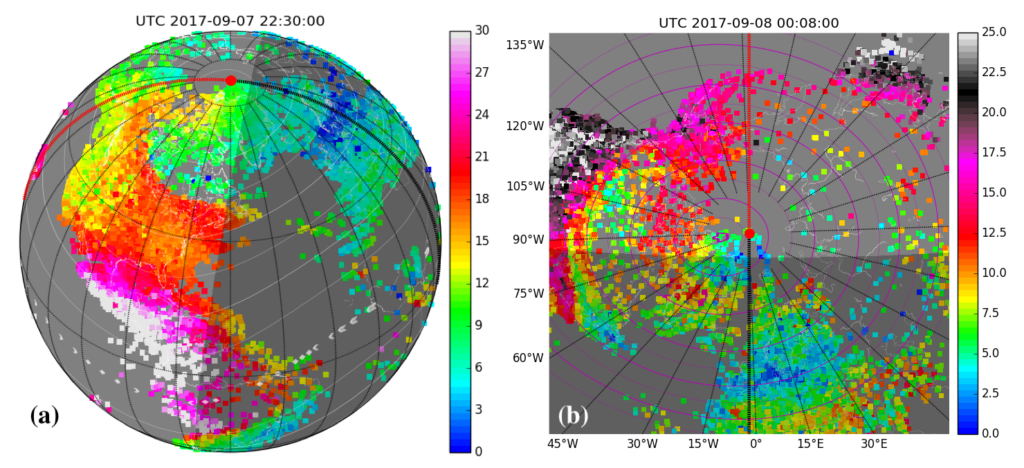
Current research includes:
- Subauroral Polarization Stream (SAPS), using unique IS radar measurement capabilities
- Storm-enhanced density (SED) plumes, the mid-latitude ionospheric trough, polar tongue of ionization (TOI), polar plasma patches, and other ionospheric plasma density gradient structures
- Ion-neutral coupling driven by SAPS and SED processes
- Geospace storm-time traveling ionospheric disturbances (TIDs)
Solar Flare and Solar Eclipse Impacts
Transient ionospheric perturbations include solar flare and solar eclipse impacts.
Dynamic celestial events such as these provide a natural way to conduct active experiments in ionospheric and thermosphere physics on a planetary scale in a way that is nearly impossible to reproduce using systems of human origin. Haystack’s Atmospheric and Geospace Sciences group is actively pursuing studies of these events to increase our knowledge of the entire atmospheric system and its variations.
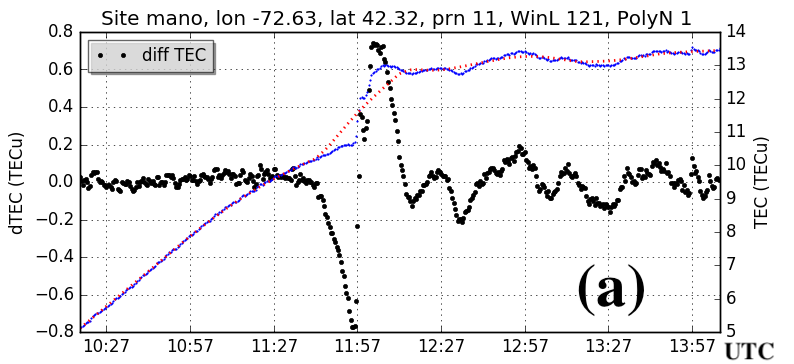
A solar flare injects a sudden and strong energy input to the sunlit upper atmosphere at a range of radiation wavelengths that are important for both ionospheric photochemistry and thermospheric dynamics. Impulsive energy inputs from flares are well known to generate sudden ionospheric density enhancements with subsequent quick time decay. Recent Haystack-led studies of solar flare response have provided direct observations that point to a previously unconfirmed set of ionospheric oscillation and traveling disturbance mechanisms associated with solar flare impacts near the sunrise terminator. Millstone Hill incoherent scatter radar measurements of ionospheric variations as a function of altitude during solar flare events provided key ionospheric plasma parameter state information that revealed significant ionospheric expansion/up-welling at the flare peak, as mass and energy were redistributed. These redistributions form an important part of space science community understanding of space weather and its impacts.
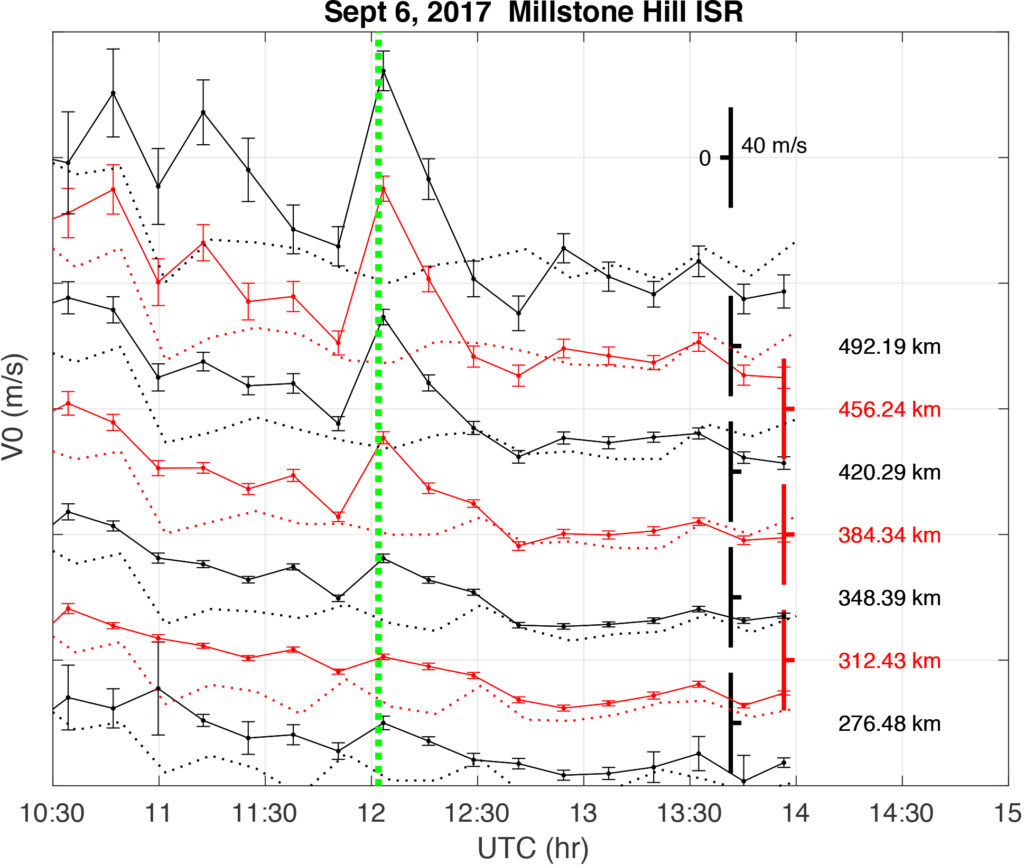
Solar eclipses provide a very different but equally fascinating transient ionospheric disturbance, with the moon’s shadow causing a large reduction in atmospheric energy input. In general, due to solar system orbital geometry, Earth experiences one or two total or partial eclipses per year at different locations and with different durations, forming a range of unique experiments. A key feature of eclipse events lies in the shadow’s supersonic motion compared to the normal sound speed set by pre-eclipse conditions in the background ionosphere. This supersonic feature has long been theoretically expected to generate atmospheric bow waves, similar to those provided in water by a fast-moving river boat. During the August 2017 eclipse over North America, we found the first unambiguous experimental evidence of these bow-shaped traveling ionospheric disturbances in a spectacular series of wave signatures that traversed over central/eastern US for about an hour, emanating from and subsequently tailing the eclipse totality region with a significant 280 m/s phase speed.
Ionospheric Climatology and Long-Term Changes
Upper atmospheric climatology, long-term trends, and lower atmospheric influences form the core background knowledge of slower time processes in both the ionosphere and neutral thermosphere. Understanding these climatologies is crucial to studies of ionosphere trends over years to decades, along with seasonal influences and their sources in first-principles physical mechanisms. This understanding is also very important to the study of space weather, upper atmospheric tides, planetary waves, and other phenomena which depend on an accurate subtraction of long-time climate in order to reveal dynamics and wave action.
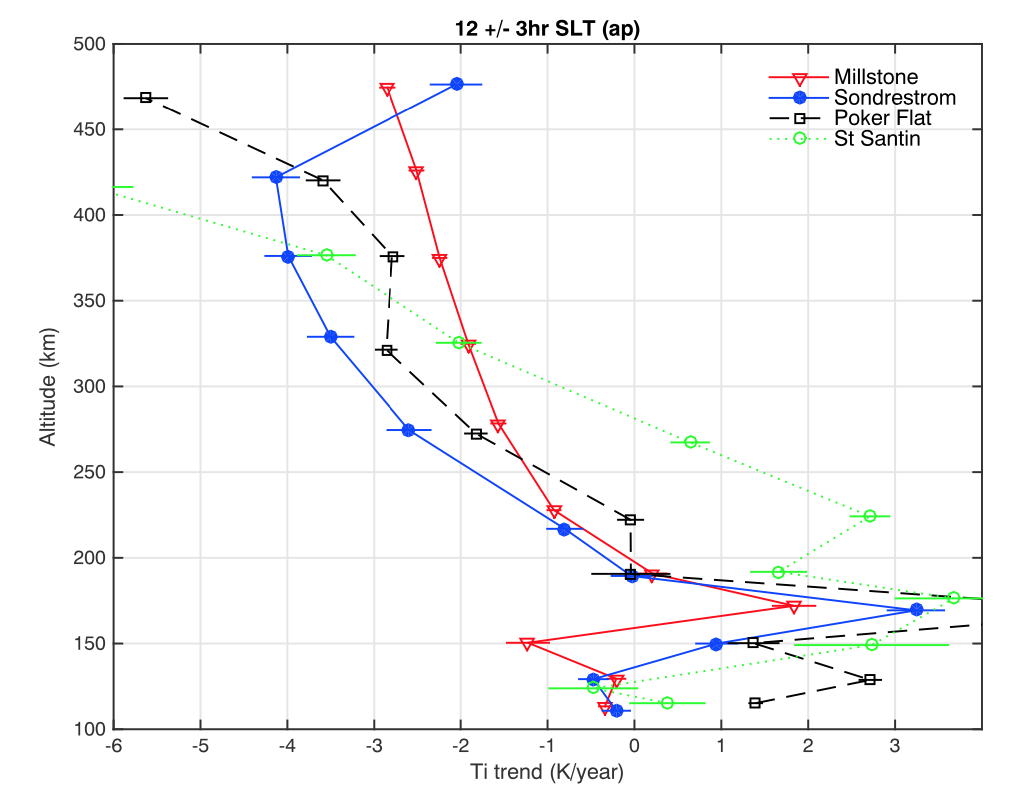
Core Haystack geospace research in this area includes several subjects:
- Ionospheric perturbations caused by tight coupling to meteorological/atmospheric processes including tidal motions, traveling atmospheric disturbances, neutral atmosphere composition changes, and multi-day planetary waves.
- Ionospheric empirical models, providing climatology and unlocking studies of the fundamental chemistry and physics of the whole atmosphere system.
- Long-term trends in the upper atmosphere. The Millstone Hill incoherent scatter radar provides a unique capability for direct long-term monitoring of the thermal status of the upper atmosphere. Studies have identified a long-term cooling trend in the ionosphere that grows in strength with height above 200km. The increasing anthropogenic greenhouse gas concentration that has led to global warming in the surface temperature contributes to this trend. However, we are in the process of identifying additional sources for the cooling, such as secular changes in Earth’s background magnetic field.
Radar Technology
Haystack scientists and engineers use radar (originally an acronym for radio detection and ranging), a system that uses radio waves to determine information about objects. Radar is essential to measure various aspects of our atmosphere. The observatory uses this technology in a number of different ways to understand our environment.
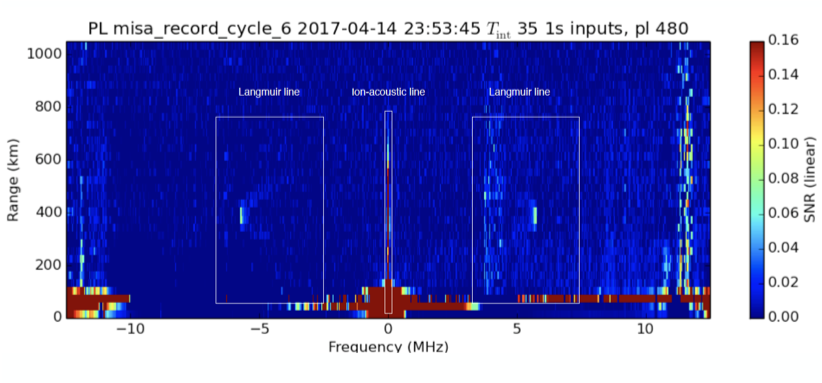
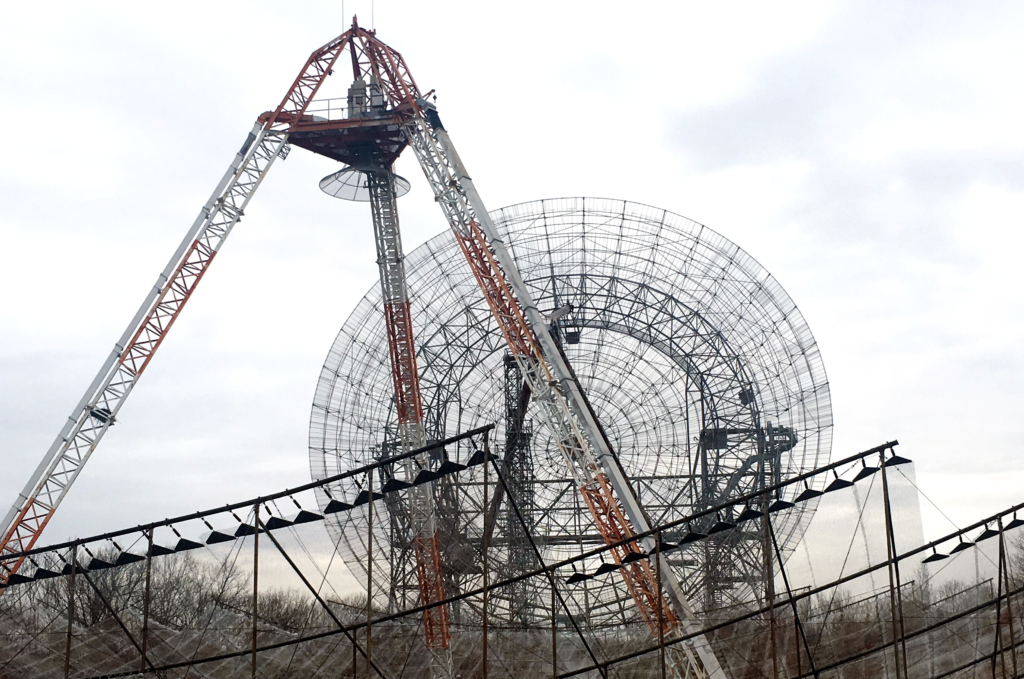
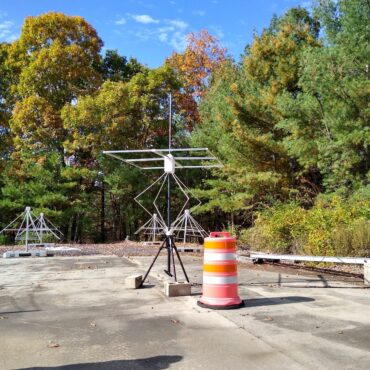
ElectroMagnetic Vector Sensor Ionospheric Sounder (EMVSIS)
EMVSIS (pronounced "emphasis") is a brand-new radar network for studying the effects of the 2024 solar eclipse on the Earth's atmosphere.
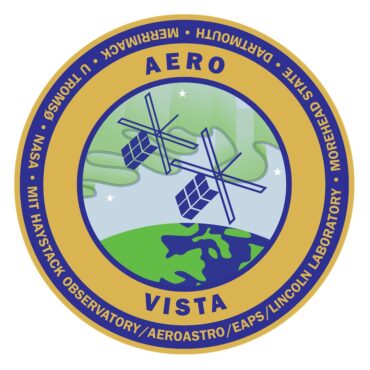
AERO-VISTA CubeSat mission
A NASA twin CubeSat mission for auroral radio science and space interferometry technology.
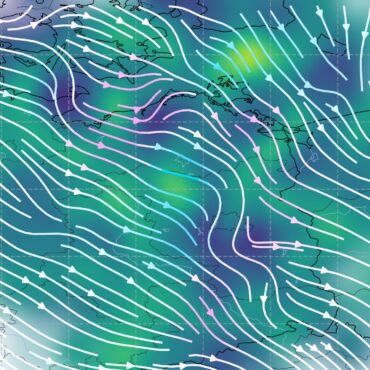
Zephyr Meteor Radar Network
A novel multiple-input/multiple-output (MIMO) radar network designed to estimate the 3D wind field in the upper atmosphere by way of meteor trail scattering.