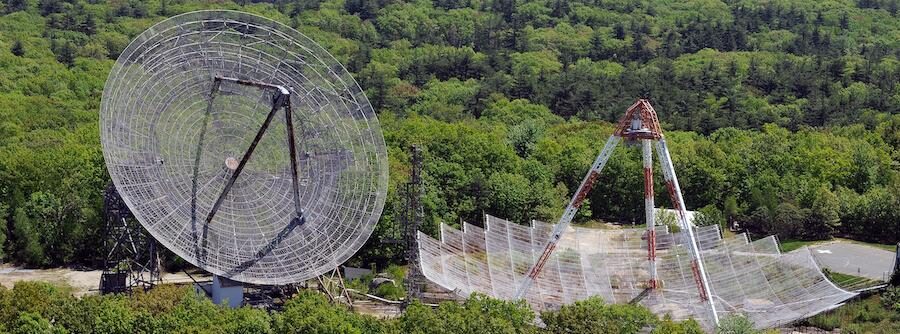
Geospace
The Atmospheric and Geospace Sciences group at Haystack conducts fundamental and collaborative scientific research into the complex and highly intercoupled near-Earth space environment using a wide variety of observational experimental data sets. These include radio (passive), radar (active), and other remote sensing tools using instruments such as incoherent/Thomson scatter radar, ionosondes, HF based convection radars (SuperDARN), optical neutral airglow observations, and in-situ satellite instruments, including small satellite platforms.
Studies of ionospheric response in both quiet and active times also involve collaborative data-model investigations using frontier whole atmosphere community models.
The Madrigal database is available at: http://cedar.openmadrigal.org/.
Spotlight Projects
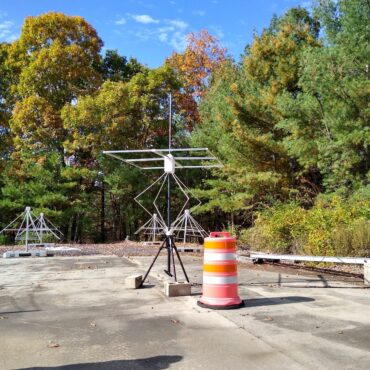
ElectroMagnetic Vector Sensor Ionospheric Sounder (EMVSIS)
EMVSIS (pronounced "emphasis") is a brand-new radar network for studying the effects of the 2024 solar eclipse on the Earth's atmosphere.
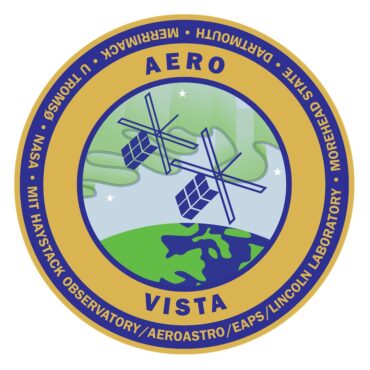
AERO-VISTA CubeSat mission
A NASA twin CubeSat mission for auroral radio science and space interferometry technology.
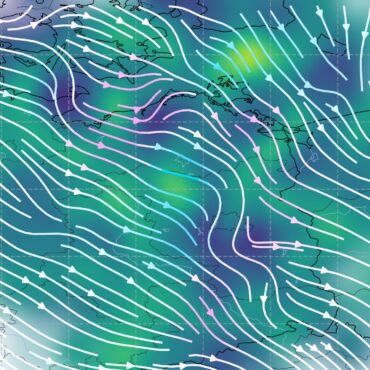
Zephyr Meteor Radar Network
A novel multiple-input/multiple-output (MIMO) radar network designed to estimate the 3D wind field in the upper atmosphere by way of meteor trail scattering.
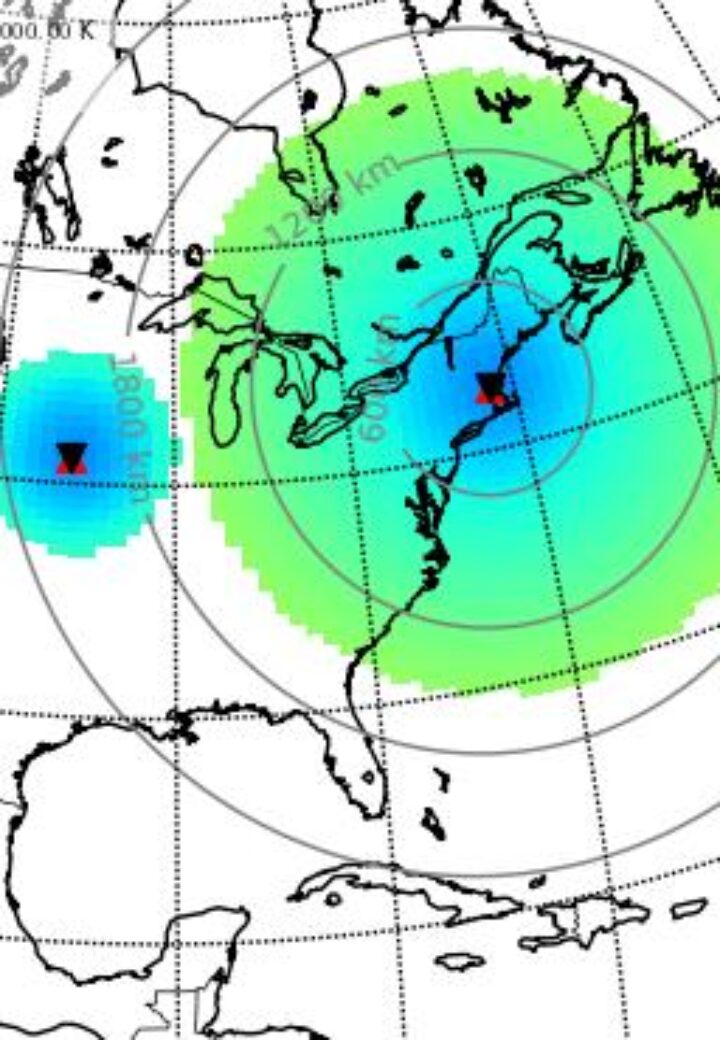